The title might grab your attention for reasons that have little to do with the content here—and that’s completely intentional. But if you’re hoping for advice on winning over your crush or unlocking the secrets to being irresistible, I hate to disappoint. This is not that kind of dating guide. This is about something far more universal, far more enduring: the science of dating… the ages of things.
Why Does Age Matter?
Why should we care about the age of anything? Simply put, understanding the ages of things around us—whether they be fossils, rocks, or historical artifacts—helps us unravel the history of the world itself. And why bother with that? Well, science is all about understanding the world around us, and the more we understand, the better the predictions we can make.
Still skeptical? Let’s consider an example that had life-or-death consequences: Cyclone Phailin in 2013. This was one of the most massive cyclones to hit India since the catastrophic Odisha cyclone in 1999. But here’s the difference—the 1999 storm claimed over 10,000 lives, while the death toll for Phailin was just 23. What changed in those 14 years? Better forecasting, thanks to advancements in meteorology. Indian authorities were able to track the storm well in advance and issue life-saving warnings, moving people out of harm’s way.
And how did we get better at forecasting? By improving our understanding of the world around us. The more data we collect, the more we know, and age determination of the things around us is key to that knowledge.
The Quest to Pin Down Time
Throughout human history, philosophers have been mesmerized by the concept of time. But for most of that history, they had very little data to support their theories. That changed with the discovery of fossils—preserved relics of life from bygone eras. These fossils became clues, helping us piece together the timeline of life on Earth.
In 1667, the Danish scientist Nicolas Steno made a breakthrough. He noticed that peculiar “tongue stones” found in Mediterranean sediments were almost identical to modern shark teeth. Steno concluded that these stones were actually ancient shark teeth that had been preserved in the rock over time. This was one of the earliest documented cases of using fossils to date Earth's history, and it opened the door to a whole new way of thinking about time.
Fair is what we see, Fairer what we have perceived, Fairest what is still in veil.
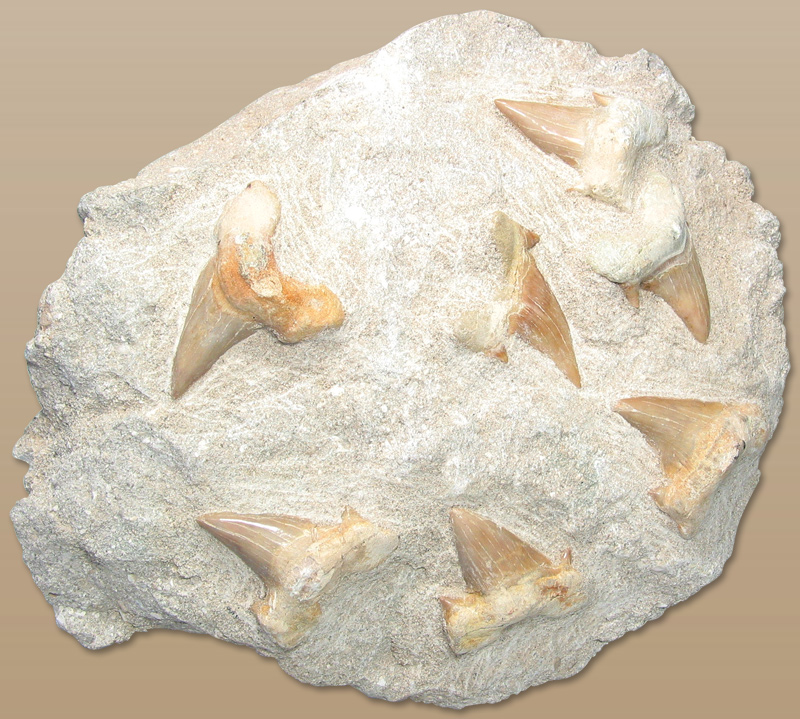
The picture above shows ‘tongue stones’ within a sedimentary rock. They got the name because earlier, people thought they were the tip of the tongues of monsters that lived under the Earth. But, Nicholas Steno rightly identified them to be ancient shark teeth and thus laid the foundation for the scientific study of fossils.
These "tongue stones" were discovered buried within layers of sediments. But what exactly are sediments? They’re naturally occurring materials—bits of rock, soil, and organic matter—that have been broken down by physical forces like wind, water, or ice, or by chemical reactions or even by biological interactions. Once broken down, these particles are transported, often by rivers, wind, or glacial movement. If you’ve ever noticed how rivers turn brown during heavy rains, that’s because they’re churning with sediments washed down from surrounding areas. As the river’s speed decreases, these sediments gradually settle to the bottom, forming layers. The same process happens all around the world.
In the case of the tongue stones, these ancient shark teeth were preserved within marine sediments. After the shark died, its body decayed, but its hard teeth were gradually buried within sediments settling to the ocean floor. Over time, these teeth fossilized into what people would later mistake for "tongue stones."
Steno’s Simple but Revolutionary Ideas
To convince people of his discoveries, Nicholas Steno even wrote a book. In it, he laid out some foundational principles of geology, two of which are surprisingly simple:
- Sediments are always deposited in horizontal layers.
- Each sedimentary layer is younger than the one beneath it and older than the one above it, as long as the sequence hasn’t been disturbed.
But why do these sedimentary layers form in the first place? Why it’s not a single continuous column of sediments from the beginning? Well, sediment deposition often occurs in cycles. Let’s go back to our tropical river example. During the rainy season, rivers carry huge amounts of sediments and deposit them wherever the water slows down, like at a river mouth. But during the dry season, there’s little sediment to transport. When the rainy season returns, a fresh layer of sediment gets deposited on top of the old one, creating a new layer. Over time, these layers stack up, preserving a record of the past.
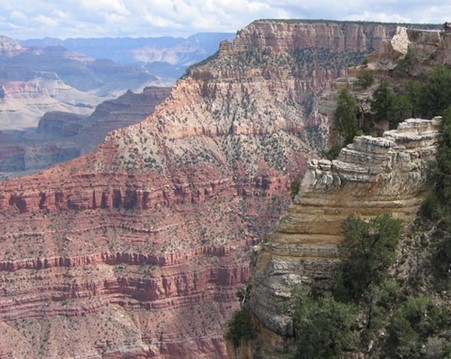
In some places, like the Grand Canyon in Colorado, these layers are incredibly distinct and visible, stretching back millions of years—a natural archive of Earth’s history.
Geologists still rely on Steno’s principles to interpret sedimentary layers, but those alone don’t tell the whole story. Sometimes there are gaps between sedimentary layers—missing chunks of time that never got recorded. These gaps, known as "unconformities," could be brief, like a dry spell between two floods, or span millions of years, caused by events like earthquakes that uplifted the land, followed by erosion that wiped away entire layers of history. These situations required an expansion of Steno’s ideas to better understand Earth’s complex past.
Enter William Smith: The Engineer who loved fossils
Over a century later, in 1793, a British engineer named William Smith, working on canal projects in southern England, stumbled upon a breakthrough. While examining the layers of sedimentary rock, he noticed that different layers contained distinct sets of fossils. This led him to propose a revolutionary idea: fossils can be used to determine the relative ages of sedimentary rocks. Smith realized that when same sequence of fossils appeared in rock layers at different locations, it meant that these layers were deposited around the same time. In other words, if two distant locations had the same fossils in their sedimentary layers, those layers must have formed during the same geological period.
This simple but profound observation became a game-changer for geology. It allowed scientists to correlate rocks from different places and piece together the puzzle of Earth's history. Smith’s work laid the foundation for a new field of science—paleontology, the study of ancient life through fossils. Paleontology didn’t just revolutionize geology; it sparked breakthroughs in other fields, too. Young Charles Darwin studied fossils, which later helped him formulate his groundbreaking theory of evolution, transforming the course of biology forever.
Clues Beyond Fossils: Magma, Faults, and Cross-Cutting Relationships
Fossils aren’t the only tools for determining the age of rocks. There are also clues hidden in how rocks are disturbed over time. Take magma, for instance. When molten rock from beneath Earth’s surface intrudes into sedimentary layers, it forms volcanic rock that cuts through the existing sequence. These intrusions are younger than the layers they disrupt. Similarly, faults—fractures in the Earth where blocks of rock shift due to tectonic forces—also slice through sedimentary layers. These faults must have formed after the sedimentary layers were deposited, making them younger than the rocks they cut through.
But what if these intrusions or faults get eroded, and new sedimentary layers are deposited on top of them? In that case, we can infer that the intrusions or faults occurred before the overlying sedimentary layers. Geologists also use this principle, known as cross-cutting relationships, to determine the relative ages of rock formations. By studying how rock layers interact with intrusions, faults, and erosional surfaces, geologists can reconstruct the sequence of events that shaped the landscape.
At the start of the 19th century, geologists began applying Steno’s and Smith’s principles, along with cross-cutting relationships, to rock formations worldwide. By the century's end, they had pieced together a global history of geologic events, culminating in one of the most important tools in geology: the geologic time scale. This time scale divides Earth’s history into distinct intervals, marked by sudden shifts in the types of fossils found in the rocks. Each boundary in the scale represents a major change in life on Earth, often due to events like mass extinctions.
The basic units of this time scale are eras, named for the types of life they represent—Paleozoic (old life), Mesozoic (middle life), and Cenozoic (new life). Each era is divided into periods, often named after the places where their rock formations were first studied. For example, the Jurassic period is named after the Jura Mountains. The Paleogene, Neogene and Quaternary periods of the Cenozoic are exceptions, meaning “old origin”, “new origin,” and "the fourth" respectively. The term "Quaternary" originates from another classification system that divided Earth's history into four periods: Primary, Secondary, Tertiary, and Quaternary. While the first three divisions—Primary, Secondary, and Tertiary—are no longer in use today, the Quaternary period remains a part of the modern geologic time scale. These periods are further broken down into epochs, with today’s modern world existing in the Holocene epoch of the Quaternary period in the Cenozoic era.
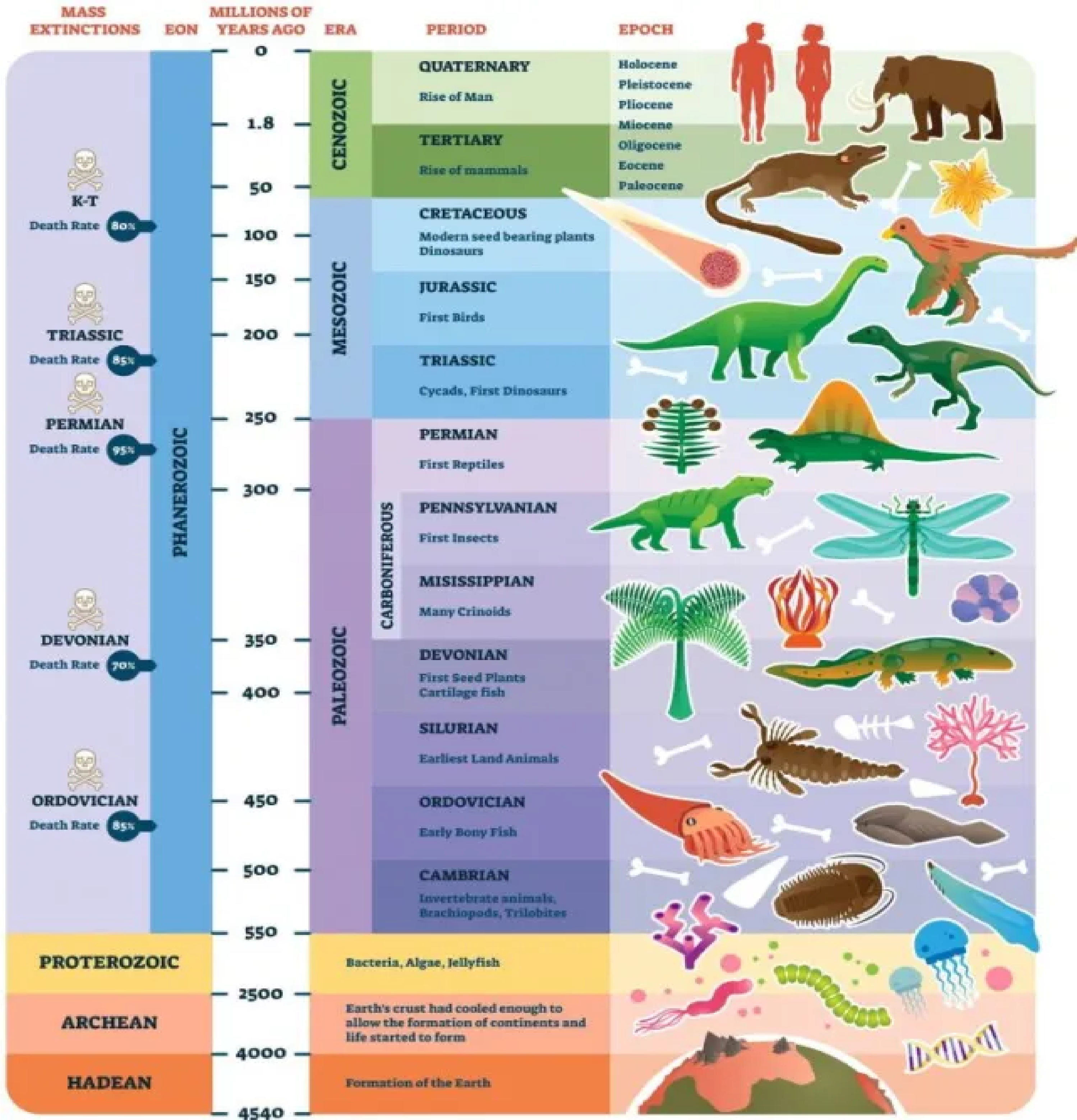
While this relative time scale told scientists what happened in sequence—what came first, what followed—it didn’t reveal the actual age of the Earth. The question of just how old our planet was remained one of the biggest mysteries of the time. There were plenty of guesses, but no solid data.
A Breakthrough in Physics: The Birth of Isotopic Dating
That all changed in 1896 when French physicist Henri Becquerel stumbled upon a discovery that would forever alter our understanding of time: radioactivity. A few years later, in 1905, physicist Ernest Rutherford made a groundbreaking suggestion: by measuring the decay of radioactive elements in rocks, we could determine their absolute age. This marked the dawn of isotopic dating—a technique that uses naturally occurring radioactive elements to accurately determine the age of materials.
Serendipity and the Discovery of Radioactivity
The story of Becquerel’s discovery is a perfect example of how chance favors a prepared mind. In 1896, Becquerel set up an experiment to explore whether X-rays could be produced by exposing fluorescent materials to intense sunlight. He wrapped a photographic plate in several layers of black paper, placed a slab of uranium salt in front of it, and exposed the setup to sunlight for several hours. His reasoning was simple: the black paper would block sunlight from reaching the photographic plate, but if X-rays were produced by the uranium, they would penetrate the paper and leave marks on the plate. And sure enough, when he developed the plate, it was fogged—exactly as expected.
But then came the twist. The next time he attempted the experiment, clouds rolled in, blocking the sunlight. Expecting clear results, he developed the photographic plates anyway—and to his surprise, they were just as fogged as before. Confused but curious, Becquerel quickly realized that the trigger of the radiation wasn’t the sunlight at all—it was the uranium itself. He had accidentally discovered radioactive decay, a process that occurs when the nucleus of an atom becomes unstable and releases energy.
Becquerel’s discovery of radioactivity would go down in history as one of the great serendipitous moments of science, showing just how much a prepared mind can comprehend when given an unexpected opportunity. This breakthrough would ultimately lead to the development of isotopic dating, forever changing our understanding of the Earth’s history.
More on isotopic dating in the next part.
To be continued...
Leave a Reply
Your email address will not be published. Required fields are marked *
Comments (0)